The endocrine system plays a key role in exercise in both acute responses and long-term adaptations. This system consists of hormones, the glands that produce them, and the receptors in the body that respond to hormones. Fitness professionals must have a basic knowledge of the endocrine system given its importance to exercise science for properly understanding the adaptations that occur in the body in response to exercise.
Introduction to the Endocrine System
โโThe endocrine system is a three-part system composed of endocrine glands, hormones, and receptors.
- Glands are scattered throughout the body and in specialized brain areas. The cells in these glands carry out functions by secreting specific chemicals called hormones.1,2
- Hormones are chemical messengers released in the blood that causes a change in the target cell when binding to receptors.1,2
- Receptors are docking molecules in the target cell that get activated when a hormone binds to it, triggering a cascade of biochemical reactions that eventually modify the cellโs function or activity.1,2
For the body to function, it requires interdependent communication and close coordination between all seven systems in the human body. The endocrine system evolved as a mechanism to communicate and regulate signals within the body.
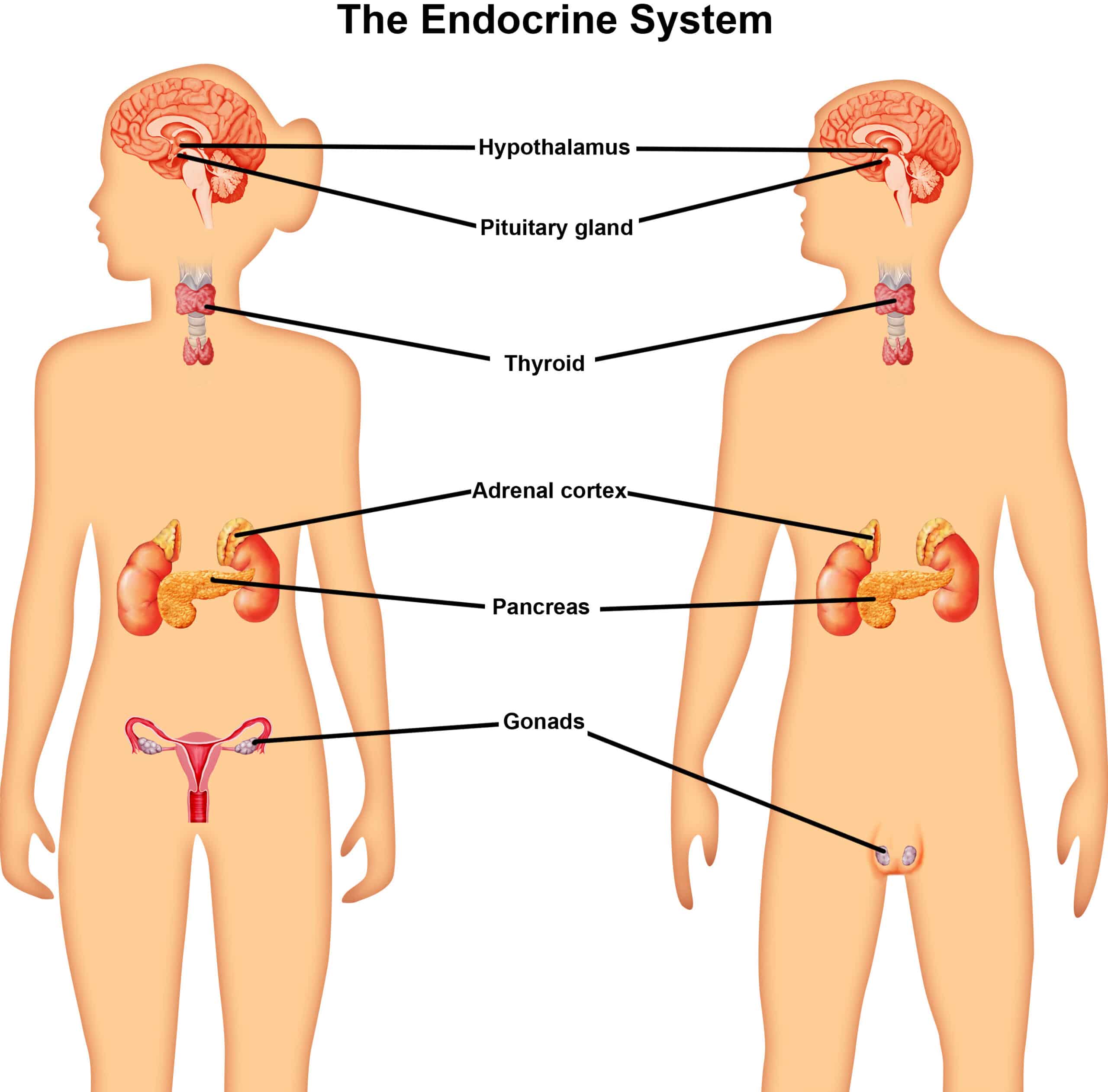
The endocrine system is a complex network of glands, hormones, and receptors that works harmoniously with the nervous system to control and coordinate energy, reproduction, growth, immunity, and behavior.1 To ensure that a constant internal environment (i.e., homeostasis) is maintained, the nervous system allows rapid transmission of information from the brain to the nerves in the body.1,2
Conversely, the endocrine system relies on producing and releasing hormones from various glands and transporting those hormones via the bloodstream.1,2 Thus, the two communication systems work in tandem to complement each other.
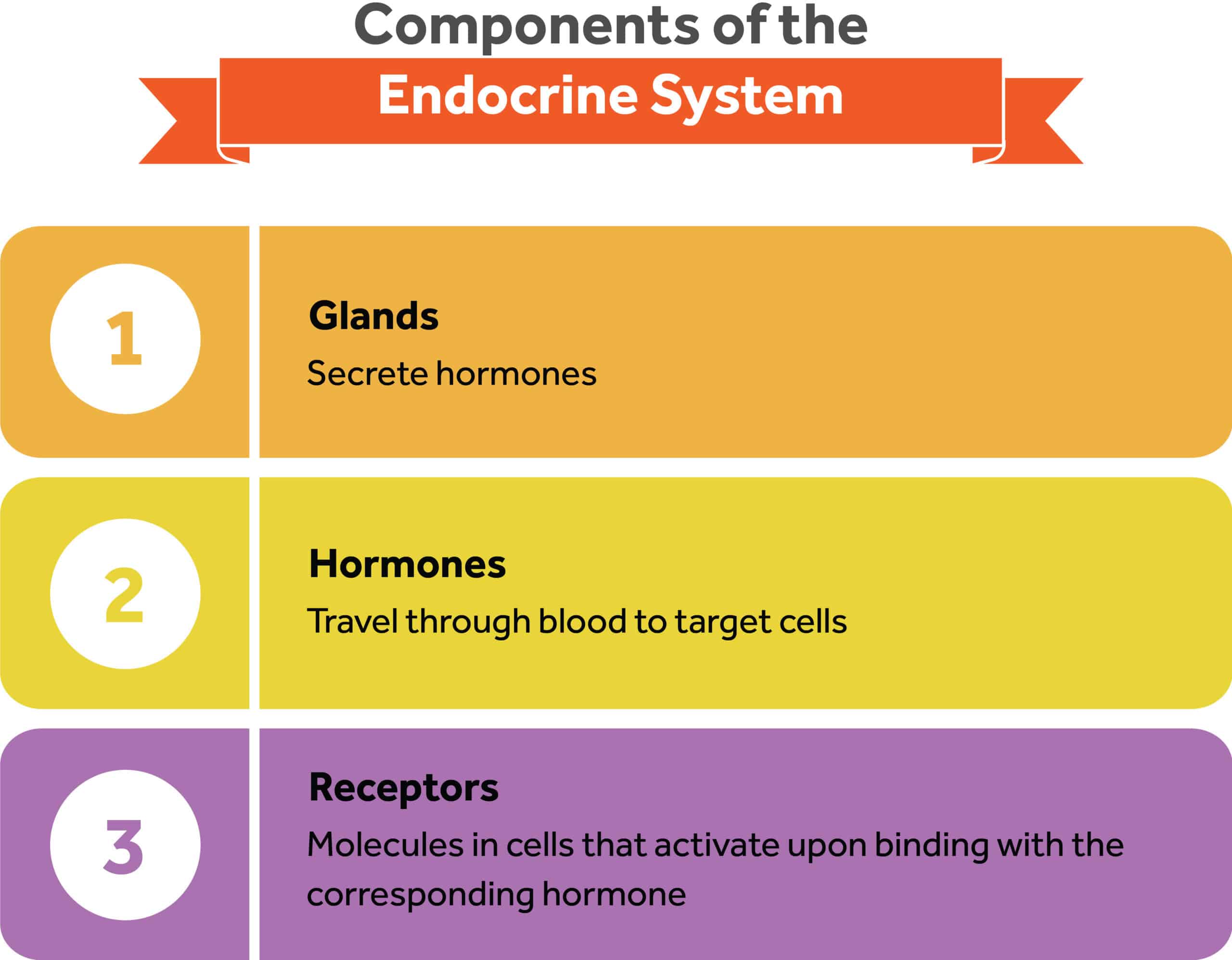
Major Endocrine Glands and Hormones
Most hormones are produced by endocrine glands. In response to a stimulus, hormones are released from the gland directly into the bloodstream until they reach the target organ.2 An example of a classical endocrine gland is the thyroid gland, which functions to synthesize and release thyroid hormones into the bloodstream.2 On the contrary, exocrine glands release hormones through a duct or opening to a body surface.3 Examples of exocrine glands include sweat glands, lacrimal glands, salivary glands, mammary glands, and digestive glands in the stomach, pancreas, and intestines.3
The following table shows the major endocrine glands and the hormones they secrete:
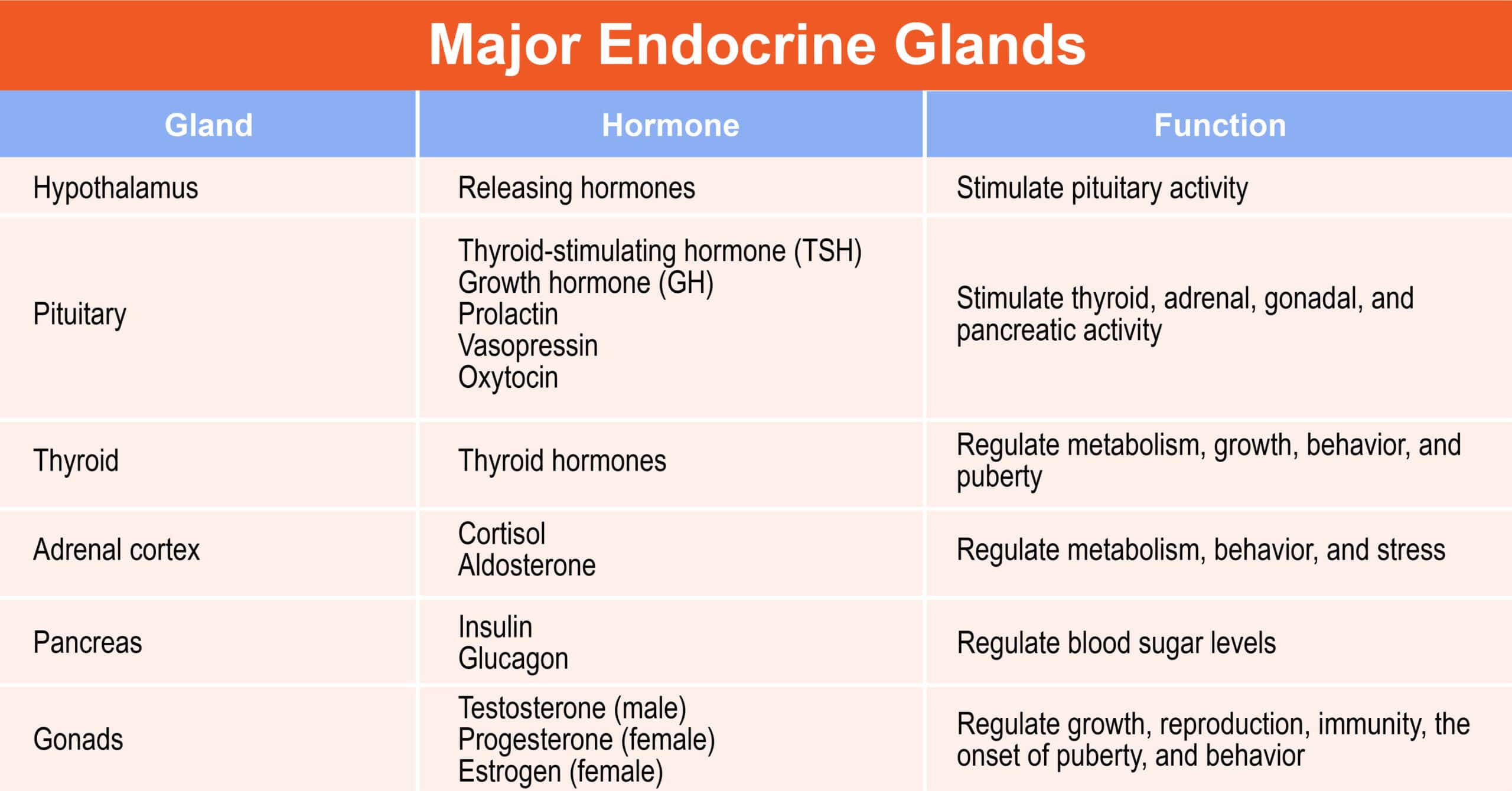
Gland | Hormone | Function |
Hypothalamus | Releasing hormones | Stimulate pituitary activity |
Pituitary | Thyroid-stimulating hormone (TSH), growth hormone (GH), prolactin, Vasopressin, Oxytocin | Stimulate thyroid, adrenal, gonadal, and pancreatic activity |
Thyroid | Thyroid hormones | Regulate metabolism, growth, behavior, and puberty |
Adrenal cortex | Cortisol, aldosterone | Regulate metabolism, behavior, and stress |
Pancreas | Insulin, glucagon | Regulate blood sugar levels |
Gonads | Testosterone (male), progesterone (female), estrogen (female) | Regulate growth, reproduction, immunity, the onset of puberty, and behavior |
Functions of the Hormones in the Human Body
Hormone Regulation
Maintaining a stable physiological equilibrium (homeostasis) requires tight control of hormone production and regulation. Hormone regulation begins in the hypothalamus, the master switchboard gland that sits at the base of the brain and is about the size of an almond.2 Below the hypothalamus sits the pituitary gland which responds to the commands of the hypothalamus.2
When the endocrine system perceives stimuli, it regulates hormones through a negative feedback loop. When an endocrine gland senses high concentrations of one hormone in the body, it changes to decrease the production of that hormone and bring a stable equilibrium in the system. This three-way communication method begins in the hypothalamus, followed by the pituitary gland until it reaches the target organ.2 When the circulating hormones have reached the target cells and levels are sufficiently high, the hypothalamus and pituitary gland cease release, thereby turning off the cascade.2 In some cases, the sensitivity of the feedback systems alters based on physiological states or stages of life. For example, in fetal development, the concentration of growth and sex hormones is the highest and culminates in adulthood when the sexual glands have acquired total reproductive capacity.4 On the contrary, positive hormonal feedback is possible in the case of ovulation in the menstrual cycle.
Behavior Regulation
Hormones play an important role in influencing the nervous system. Over time, they have evolved to influence body systems in paths that elicit the appropriate behavior or social cue. In all mammals, the interacting components of the behavioral system include
- Inputs (sensory systems)
- Integrators (central nervous system)
- Outputs (tissues and organs)
Because endocrine glands produce hormones that can enter target cells and change gene expression, specific behaviors can occur in the presence of signals, stimuli, and receptor interactions.2
For example, estrogens, the female hormones associated with reproduction, growth, and onset of puberty, show similarities with the regulation of female sexual behaviors. Interestingly, the reciprocal relation can occur where behavior affects hormone concentrations. For example, high testosterone concentrations are associated with aggression, while cortisol and serotonin act antagonistically to reduce the effects of testosterone.5
As previously discussed, the hypothalamus is the primary center for communication between the nervous and endocrine systems. One way hormones regulate mood and behavior is through the hypothalamus-pituitary-adrenal axis (HPA-axis).2 For example, HPA axis overstimulation and chronically high cortisol levels are associated with depression, anxiety, mood swings, and irritability.6
Another way the brain can regulate emotions is by releasing certain chemicals called neurotransmitters. Neurons release neurotransmitters to communicate between different brain areas and influence human moods, emotions, and behavior.7 Four main chemicals are associated with behavior regulation, including serotonin, dopamine, adrenaline, and oxytocin.
- Serotonin is the neurotransmitter responsible for pleasure, happiness, and stability.7
- Dopamine is the neurotransmitter responsible for reward, joy, and happiness.7
- Norepinephrine is the neurotransmitter responsible for fear and anger emotions that trigger a โfight or flightโ response.7
- Oxytocin is the neurotransmitter responsible for love and attachment.8
Sex-Related Differences
Humans, like most animals and plants, present with one obvious anatomical difference, the existence of two biological sexes. According to biologists, sex is the trait that differentiates males or females based on reproductive and sexual organs, chromosomes (XX for female and XY for male), gene expression, hormone levels, and physiological features.9 Gender refers to the range of socially constructed roles, behaviors, expressions, and identities used to categorize male and female differences.9
Reproductive Organs
The reason why two distinct sexes, males and females, are commonly necessary for life to reproduce is one of the oldest biological enigmas. One prominent theory is that sex evolved to produce variation, an adaptative trait that gives organisms an advantage in changing environments.10 The main distinction between males and females is the way DNA is packaged into the sex cells that make new organisms, called gametes.2 The female sex is capable of producing large gametes (ovules), whereas the male sex produces small gametes (spermatozoa).2
Gonads and Sex Hormones
The second characteristic differentiating males and females are the presence of endocrine glands and sex hormones. First, gonads are specialized reproductive glands that produce germ cells.2 The ovaries are the female gonads that produce ova, and the testes are the male gonads that produce spermatozoa.2 Second, sex hormones synthesized by gonads play an important role in physiological distinctions between males and females.2 While males and females generally have the same hormones (i.e., estrogens, progesterone, and testosterone), their production sites, blood concentrations, and interactions with different organs are different.11 Males predominantly produce testosterone and lesser amounts of estrogen and progesterone.2 Female ovaries mainly synthesize estrogen and progesterone and produce lesser amounts of testosterone.2
In the last decade, medical advancements have broadened the understanding of the influence gender has on both human physiology and the pathogenesis of diseases. The most common anatomic-physiological difference between male and female athletes is that males commonly present with stronger bones, greater muscle mass and strength, and greater aerobic capacity. In contrast, females exhibit less muscle fatigue and faster recovery during endurance exercise.12 Beyond that, conditions such as thyroid disease, diabetes mellitus, osteoporosis, obesity, and sarcopenia show sex-specific patterns in disease prevalence, pathogenesis, outcomes, adverse events, and responses to medical treatment.13 Thus, when working with individuals with medical conditions, it is crucial to tailor exercises considering sex and gender in every disease element, from the causes to the treatment.
Hormones and Exerciseย
Several environmental stressors affect an organism’s biology and endocrine system. Engaging in strenuous forms of exercise and training for sports competitions are examples of situations that trigger a stress response in the body and impact hormone homeostasis. This section will focus on understanding the role testosterone, estrogen, GH, insulin, glucagon, catecholamines, cortisol, and thyroid hormones play in response to exercise.
Testosterone
Testosterone (T) is the main androgenic steroid hormone, abundantly produced in the testes and in lesser amounts in the ovaries and adrenal cortex.2 As an androgen, its primary role is to stimulate the development of male characteristics.2 The specific functions of testosterone vary during different developmental stages. For example, during fetal development, testosterone is most elevated to support the growth of male genitalia.2,14 Until then, males and females have similar amounts of testosterone production. However, during puberty, there is an approximately 30-fold increase in testosterone production in males, resulting in changes in mood and behavior, and anatomical traits such as height, deepening of the voice, growth of facial, pubic, axillary, and body hair; and increase in muscularity and strength.2, 15 As a result of this higher testosterone production, boys gain considerably more lean body mass than girls.16 In adult life, testosterone maintains libido and regulates sperm production.2
In addition, testosterone plays an essential role in regulating muscle protein synthesis and muscle hypertrophy. New research suggests that chronic increases in testosterone levels can significantly increase hypertrophy and strength, whereas decreases in basal testosterone levels result in the opposite effect.17 For both sexes, the typical pattern of testosterone release commonly follows a diurnal rhythm, with peak concentrations in the morning and a progressive decline over the day.18 In women, testosterone concentrations can also fluctuate as a function of the menstrual cycle.19
Estrogen
Estrogen (E) is a steroid hormone that virtually exists in the body as estradiol and is associated with the female reproductive organs.2 In females, estrogen is mainly produced in ovarian androgens, whereas in males, testes produce only 20% of circulating estrogens, with the rest deriving from peripheral organs like adipose, brain, skin, and bone, which convert testosterone to estrogen.20
The main function of estrogen is to coordinate the normal development and functioning of the female genitalia and breasts.2 Serum estrogen concentrations are highest during female pregnancy than during the rest of the female life cycle. In practice, estrogen is a reliable indicator for assessing fetus injury and early prevention of chronic placental insufficiency.21 During puberty, estrogen concentrations increase to stimulate the growth of the uterus, breast, and vagina; coordinate fat deposition and distribution in the body; regulate the pubertal growth spurt and cessation of growth at adult height, and control the development of secondary sexual characteristics.2 In adult women, estrogen controls the menstrual cycle, pregnancy, and lactation and maintains female libido.2 Later in life, the ovaries will stop producing estrogen (i.e., menopause) and are accompanied by symptoms such as changes in monthly cycles, hot flashes, sweating, heart palpitation, increased irritability, anxiety, depression, and brittle bones (i.e., osteoporosis).2
While the effects of aging on women are associated with several negative outcomes, including a decline in sex hormone production, an increase in injuries, and muscle wasting, estrogen is known to have a protective effect on musculoskeletal function. There is evidence to suggest that estrogen stimulates muscle repair and the regenerative processes by improving the structure and function of musculoskeletal tissues like muscles, tendons, and ligaments, increasing muscle mass, strength, and collagen content.22 In turn, these effects influence muscle contractile properties and aid in post-exercise muscle damage.22 During exercise women generally exhibit a greater increase in serum concentrations of estrogen than males.23 As a result, female athletes that permanently show low levels of female sex hormones, accompanied by menstrual cycle perturbations, are more prone to stress fractures and ligament injuries.24,25,26
Growth Hormone
Growth Hormone (GH) is the most abundant hormone produced by the pituitary gland.2 As the name implies, GH regulates the growth and development of reproductive organs, adipose tissue, connective tissue, endocrine glands, muscle, and bones.2 The specific functions of growth hormones vary during different life stages of development. GH levels peak in early childhood to support tissue growth and decline afterward.2 Nevertheless, GH production in adult life is still important as its commonly associated with symptoms of aging.2 In addition, GH gets released by stress, low blood sugar levels (i.e., hypoglycemia), strenuous exercise, and deep sleep. Ultimately, GH plays an important role in macronutrient metabolism as seen below:
- Glucose: GH lowers uptake by muscle cells, raising glucose levels in the blood and promoting glucose production from non-glucose molecules (i.e., gluconeogenesis) in the liver.2
- Amino acids: GH enhances the uptake of amino acids from the blood into cells and incorporates them into proteins.2
- Fat: GH stimulates the breakdown of lipids (i.e., lipolysis) and lipid oxidation in adipose tissue, suppresses glucose uptake, and consequently increases plasma glucose.2,23
GH is involved in development and maturation and is associated with skeletal muscle growth and function. Some research findings suggest that the anabolic action of GH is mediated by circulating insulin-like growth factor 1 (IGF-1) that comes from the liver and inhibits protein breakdown.27 Another way is by enhancing muscular and extra-muscular sites and increasing muscle mass without affecting contraction or muscle fiber composition.27 However, the literature on growth hormones contributing to tissue growth and strength remains controversial.
Additionally, there is a lack of consensus regarding sex-related differences in growth hormone response to exercise. So far, most research studies report similar results between both sexes in which men and women experience an increase in growth hormone levels in response to exercise.27 The only difference has been seen in the duration of GH release.23 For example, females experience GH peaks sooner than males, whereas men sustain longer responses.23 The attributable factors to this response are the lack of testosterone in women and the compensatory mechanism of having higher concentrations of resting basal level of GH and estradiol.23 Ultimately, the sex differences in growth hormone response to exercise immediately impact blood glucose control.23
Insulin
Insulin is one of the main hormones produced in the pancreas and the only blood-lowering hormone in the body.2 It is located in the beta cell of the Islets of Langerhan and is released to lower blood glucose levels after ingestion. Insulin plays an important role in all storage forms of energy and works on target organs specializing in energy storage, such as the liver, muscles, and adipose tissue as described below:
- Glucose: Insulin stimulates glucose uptake into cells, glucose breakdown, and inhibits gluconeogenesis.2
- Protein: Insulin promotes amino acid transport and protein synthesis in muscle cells, and inhibits gluconeogenesis in the muscles.2
- Fat: insulin increases fat synthesis in the liver and adipose tissue, and inhibits glycerol breakdown which also can serve as a starting material for gluconeogenesis.2
Insulin release is regulated by various factors including eating patterns, and hormones such as GH, glucocorticoids, and thyroid hormones.2 The influence of exercise on insulin has long been studied as an example of favorable adaptive change from two opposing metabolic regulatory forces. While insulin is an anabolic hormone that is secreted to increase glucose and fat storage after eating, exercise is a condition that suppresses and oxidizes the fuel storage effects of insulin.28
During exercise, serum insulin concentrations decrease by inhibiting the release of insulin from the pancreas.28 In turn, glucose uptake from glycogen reserves stored in muscles and the liver increases and produces ATP to fuel contracting muscles.29 As a result, blood sugar levels decrease up to 24 hours or more after a workout resulting in higher sensitivity to insulin.30
As an anabolic hormone, insulin stimulates the cellular pathway that regulates muscle growth. For example, patients with uncontrolled diabetes and type 2 diabetes are known to have a relative deficiency of muscle mass and quality, presumably due to insulin resistance.31 In one study, insulin administration in diabetic patients induced the expression of two muscle growth factors: MyoD, and myogenin.32 However, most studies showing positive associations are only seen in animal studies, and whether it directly stimulates muscle protein synthesis in humans remains unclear. 28
Insulin response to exercise is also highly sensitive to sex differences. Males preferentially use glucose as primary fuel during exercise, whereas females use fat as the major energy source.31 One suspected explanation for this theory is that women are more efficient at conserving energy and storing fat, based on body fat mass and other anatomical characteristics.33 Ultimately, engaging in physical activity stabilizes blood glucose levels during and after the training session, supporting the evidence that exercise improves insulin resistance in individuals with Type 2 diabetes.
Glucagon
Glucagon is the second most prominent blood glucose-regulating hormone in the body. Produced in the alpha cells of the Islets of Langerhans in the pancreas, glucagon works to counterbalance the actions of insulin.2 Its main role is to raise blood glucose levels during fasting, exercise, and hypoglycemia conditions and oppose the effects of insulin mainly in the liver as shown below:
- Glucose: Glucagon promotes glycogen breakdown and formation of glucose from non-glucogenic sources through the gluconeogenesis pathway in the liver.2
- Protein: Glucagon increases amino acid catabolism in the liver, uptake of amino acids by skeletal muscle, and increased excretion of free amino acids.2
- Fat: Glucagon stimulates the fat breakdown of triglycerides into fatty acids and glycerides for energy utilization which also can serve as a starting material for gluconeogenesis.2
As a catabolic hormone, glucagon’s role is to suppress skeletal muscle protein synthesis, irrespective of sex differences. During exercise, glucagon stimulates the liver to break down glycogen. Then, new glucose production from non-glucogenic precursors increases blood glucose, leading to hyperglycemia.34 However, arterial glucagon during exercise is incrementally delayed and dampened compared to the increase in glucose released from the liver.34 Depending on the duration and intensity of exercise, arterial glucagon may not increase.34 As energy demands from the muscle increase, glucose is released from the muscle in a glucagon-dependent manner.34
Catecholamines
Catecholamines are physiologically active compounds that act both as neurotransmitters and hormones.35 The adrenal glands are responsible for releasing dopamine, norepinephrine, and epinephrine (adrenaline) in response to physical or emotional stress. This is also known as the body’s “fight-or-flight” response.35
The significance of circulating catecholamines and their possible roles at birth is used to support neonatal adaptation.36 During early development and birth, plasma catecholamine concentrations are remarkably higher than those in adult life, primarily to maintain glucose supply to the heart and brain and to prepare the lung for ventilation.36 Regarding sex differences in catecholamine levels, young females exhibit a lower adreno-sympathetic maturity than males. However, both sexes reach full maturity of the adrenergic sites near the fifth year of age.37 In adult life, women tend to secrete and clear epinephrine out of the body at lower levels than men. Nevertheless, this change is not dependent on adrenergic maturity but rather is from the increased rate of catecholamine removal.37
Exercise increases catecholamine concentrations in athletes, specifically adrenaline and noradrenaline in a sex-dependent manner.38 One study found that men’s epinephrine and norepinephrine levels were higher throughout the training period than those of women.38 This occurs by stimulating hepatic glycogenolysis and gluconeogenesis, increasing blood glucose levels during activity, and potentially decreasing glucose levels post-exercise when glycogen stores are being replenished.23 Therefore, the catecholamine spike seen in men who exercise could have resulted in a higher mobilization and utilization of muscle glycogen. Ultimately, studies on women remain scarce and the effects of exercise on catecholamine response remain to be specified.
Cortisol
Cortisol, also known as the stress hormone, is a steroid hormone synthesized from cholesterol by the adrenal cortex and regulated by the hypothalamus-pituitary-adrenal (HPA) axis.39 As one of the principal glucocorticoids released from the adrenals, cortisol has many functions in the body and can affect nearly every organ system. The primary functions of cortisol include mediating the stress response, regulating carbohydrate, protein, and lipid metabolism, inflammatory response, and immune function.39
Cortisol is a hormone secreted to regulate the 24-hour cycles of the body’s internal clock, also known as circadian rhythms.40 The secretion is lowest during the early night, increases several hours before awakening, and peaks in the morning within 30โ45 min after awakening.40 In addition, the cortisol awakening response (CAR) is a separate process that works in harmony with the body’s circadian rhythms, and it’s associated with the anticipation of stressors for the upcoming day.40
Like other endocrine hormones, cortisol levels play a role in the stages of growth and development. During pregnancy, maternal cortisol levels increase by two to four times to support neural development and fetal growth.41,42 Throughout puberty and adolescence, cortisol concentrations increase significantly to function as a synchronizer for the entire circadian system.43 Additionally, cortisol production stabilizes between ages 20 to 60 and progressively increases after 60 years.44
Cortisol is released during a โfight or flightโ response to optimize bodily functions and improve alertness in physically or psychologically demanding events. First, it mobilizes energy by releasing glucose from its storage sites and raising blood glucose levels.39 Second, glucose uptake decreases in the muscle and adipose tissue and increases glycogenesis and gluconeogenesis in the liver. This effect results in muscle growth inhibition.39 In adipose tissues, cortisol increases lipolysis. Lipolysis is a catabolic process that releases glycerol and free fatty acids.40 Lastly, cortisol facilitates the pancreas to decrease insulin and increase glucagon and enhances the activity of epinephrine, and other catecholamines.39
It’s theorized that repeated exercise can habituate or sensitize the physiologic stress system or HPA axis.45 While most studies show that exercise intensity correlates with cortisol release above resting levels, high cortisol levels can inhibit protein synthesis resulting in skeletal muscle protein breakdown.46 However, serum cortisol levels decrease shortly after exercise and suppress cortisol release at night, subsequently diminishing cortisol responses to psychosocial stressors.45 Ultimately, continuously engaging in physical activity mediates the stress response and endorphin release resulting in feelings of relaxation and mood improvement.
Thyroid Hormone
The thyroid hormone is composed of two structurally related hormones produced by the thyroid gland: thyroxine (T4) and triiodothyronine (T3). Thyroid hormones increase the metabolism of almost all body tissues.2 This includes heat regulation, energy metabolism, growth and maturation, and development of the central nervous system, tissues, and bones.2 Ultimately, the thyroid gland is virtually responsible for maintaining and regulating all body systems required for life, including the nervous, cardiovascular, and gastrointestinal systems.
As the regulator of several systems, the thyroid hormone significantly contributes to the body’s energy metabolism in skeletal muscle functioning. First, thyroid hormones increase the basal metabolic rate and favor metabolic reactions such as lipid and carbohydrate breakdown and anabolism of proteins.2 Second, thyroid hormones regulate changes in the demand and synthesis of ATP in the skeletal muscle.47 Third, thyroid hormones act on several gene targets that result in the expression of genes responsible for fast-twitch fibers, muscle development, homeostasis, and regeneration.47 Finally, thyroid hormone availability influences contractile and relaxation reflexes of the skeletal muscle.47
The influence of exercise on thyroid function increases the release of thyrotropin-releasing hormone (TRH) that stimulates the secretion of TSH in the pituitary gland. While basal metabolic rate increases with exercise and provides several benefits in different body systems, strenuous exercise may be associated with transient alterations in thyroid hormones.48 For example, an underactive thyroid (hypothyroidism) reduces cardiopulmonary function, fatigue, muscle stiffness, and exercise intolerance.47 In contrast, hyperthyroidism caused by increased thyroid gland function manifests as weight loss, heat intolerance, diarrhea, fine tremor, and muscle weakness.47
Interestingly, thyroid diseases affect individuals in a sex-dependent manner, with a prevalence approximately ten times higher in women than men.49 For athletes, these symptoms can negatively impact their ability to effectively train, compete, and recover. For example, a side effect of overtraining in female athletes is secondary amenorrhea which can lead to thyroid dysfunction.49 In addition, athletes with low growth hormone, cortisol, and thyroid-stimulating hormone levels are at risk for diminished tissue growth, repair, and other neuromuscular disorders.47 Nevertheless, consistency in training is not associated with thyroid function improvement among individuals with thyroid disorders. While there is a decrease in serum concentrations of TSH, T3, and T4 in periods of increased physical activity, these changes are minor and have the potential for physiologic adaptation.48 Therefore, individuals with thyroid alterations should seek advice and pharmacological treatment from a medical professional and pursue exercise training as a lifestyle modification rather than treatment.
Acute and Chronic Adaptations to Exercise
As previously seen, hormonal adaptations naturally result from engaging in physical activity. This section will examine the acute and chronic responses of the endocrine system from various forms of exercise, taking a closer look at the effects of modality, duration, and intensity of a training session.
Acute responses are those that occur immediately after training and in the subsequent hours and days. These effects may dissipate relatively quickly.
Chronic responses involve long term changes associated with resistance training. These changes are more long lasting, although inactivity can result in reversal.
Testosterone
Testosterone concentrations are positively associated with exercise modality and duration. Total testosterone concentrations acutely increase in most men and young women during a training session, while no changes appear in middle-aged and elderly women.50 While this mobilization of testosterone is brief, the effects of testosterone can last from 15 minutes to several hours and support favorable adaptive responses to muscle gain.17 Interestingly, the acute elevation is higher in resistance-trained men than in endurance-trained men.50 Additionally, one study assessing weight distribution found that testosterone and muscle strength are higher if lower-body exercises are performed before upper-body training.51 Ultimately, moderate-load, high-volume training with short rest periods produces a more significant testosterone response than high-load, low-volume training with long rest periods.51
Chronic testosterone changes during and post-resistance training have been inconsistent in the literature on both men and women. While some studies have demonstrated a chronic increase in basal testosterone, others have failed to ๏ฌnd an adaptation to regular resistance exercise.50 For example, significant elevations have been reported in pubertal boys, whereas no difference exists in resting concentrations between untrained and elite athlete females.50 On the contrary, most reports show that resting concentrations of testosterone decrease when there are reductions in volume and intensity of the training period, leading to the speculation that perhaps higher volumes are needed to alter resting levels of testosterone.50 Ultimately, chronic adaptations from resistance training are associated with increases in lean mass and strength.
Growth Hormone
Growth Hormone acutely increases after resistance training in both men and women. Particularly, concentrations are elevated through 30 minutes post-exercise in both sexes, although the resting concentrations of GH are higher in women.52 Additionally, programs of moderate to high intensity, high volume, and short intervals are most successful with the responses compared to conventional programs using high loads, low repetitions, and long intervals.50 Chronic concentrations of GH are not affected by traditional resistance training programs as seen in both men and women.50 This data is also supported by research demonstrating similar concentrations in elite Olympic athletes and strength athletes compared with non-trained individuals.50
Insulin
Insulin is affected by blood glucose concentrations and dietary intake. Not taking protein or carbohydrate supplementation before, during, or post-acute resistance training show a significant decrease in insulin.50 During aerobic exercise, insulin levels decrease and counter-regulatory hormones (i.e., glucagon, cortisol, growth hormone, norepinephrine) increase to keep blood glucose levels steady.53 Whereas in anaerobic exercise, insulin levels do not fall as much as during aerobic exercise, and glucose levels tend to rise secondary to counter-regulatory hormones.53 After training, insulin sensitivity also increases and remains high for about 24 hours, and with prolonged strenuous exercise, insulin sensitivity increases for up to 48 hours.53
Insulin adaptations can also be related to insulin-like growth factors (IGF-1) given their importance in protein synthesis during resistance training and muscle hypertrophy.50 However, the acute responses of this hormone remain unclear in the literature. In general, acute elevations following short resistance training periods may be delayed until GH-stimulated synthesis and secretion from the liver take place.50
Chronic adaptations are often evaluated in the context of IGF-1 rather than pancreatic insulin hormone. Similarly, IGF-1 shows no chronic adaptations in response to resistance training.50 In general, resistance-trained men had higher resting IGF1 than non-trained men.50 In women, higher serum elevations of resting IGF-1 during high-volume training are seen in studies with longer protocols.50 It also appears that the volume and intensity of training are important for chronic adaptations of IGF1, as one report showed reductions in IGF1 during strenuous training.50
Catecholamines
Catecholamine changes after exercise have mainly been studied in the context of acute adaptations. An acute bout of resistance training increases plasma concentrations of epinephrine, norepinephrine, and dopamine.50 In addition, before a strenuous exercise, the plasma concentration of catecholamines also increases.50 Similar effects also occur when endurance-trained individuals are compared to sedentary subjects in response to the same relative intensity exercise. This phenomenon is described as the “sports adrenal medulla”, a long-term adaptation of an endocrine hormone to physical training.54 However, chronic adaptations remain unclear, although research findings show that training reduces the catecholamine response to resistance exercise.50
Cortisol
Cortisol concentrations significantly increase during an acute bout of resistance training in both sexes. The research shows that this response is independent of training status in the adolescent population.50 Particularly, metabolically demanding protocols of moderate to high intensity, high volume, and short rest periods report the greatest acute cortisol response.50 Similarly, the number of sets per exercise appears to influence responses as well, with four to six sets of resistance exercises resulting in significant results compared to two sets.50
Cortisol resting levels are a reflection of long-term training stress. Chronic resistance training is not a factor that produces consistent patterns of cortisol secretion either. The evidence consensus determines that acute cortisol responses are associated with metabolic stress, whereas chronic adaptations are related to protein metabolism and tissue homeostasis.50
Other Hormones
Estrogen
Estrogen concentrations acutely increase in most women after endurance and resistance exercises and usually decrease by the 30-minute recovery.55 In premenopausal females, similar results exist.56 Another study assessing postmenopausal females found that anaerobic exercise significantly improved estradiol level and lean mass than aerobic exercises, despite having more than twice as many aerobic sessions throughout the study.57 The effects of acute estrogen release may relate to reducing exercise-induced muscle damage and improved recovery.58 Furthermore, inconclusive data remain on chronic responses to exercise and training on estrogen.
Glucagon
Acute bouts of exercise can lower blood glucose concentration for 2 to 48 hours post exercise and improve insulin sensitivity for up to 72 hours after cessation of any given exercise bout.53 When plasma glucose declines, the sensitivity of the liver to glucagon also improves.53 In response to prolonged exercises, the decrease in insulin and increase in glucagon also attenuates.53 Additionally, acute aerobic exercises show significant increases in glucagon concentrations irrespective of metabolic state.53
Thyroid Hormones
Exercise intervention studies report that thyroid hormone levels decrease in response to resistance and endurance training.59 Several analyses indicate that physical activity modulates both circulating TSH and T4 and the magnitude of TSH response to lower T4 levels.59 At low levels of T4, physically active adults appear to produce less TSH.59 Previous studies also reported that aerobic exercise increases total serum T3 and T4.60 Consequently, moderate-intensity exercise can increase T4 concentration in the blood.61 Aerobic exercise is associated with a progressive decrease or an improvement in serum thyroid stimulating hormone (TSH).62 On the contrary, 12 weeks of aerobic exercise show insignificant changes in the plasma level of TSH, T3, and T4 hormones among sedentary women.63
Summary
The endocrine system includes hormones (estrogen, testosterone, etc.), the glands that produce them (hypothalamus, pituitary, etc.), and the receptors in the body that respond to hormones. Hormones have a strong role in influencing the body’s nervous system and exercise stimulates hormones dues to system stressors for both men and women, although there are hormone differences between the genders due to physical differences.
References
- Endocrine system – chemicals – environment – European commission. https://ec.europa.eu. Accessed November 9, 2022.
- Hiller-Sturmhรถfel S, Bartke A. The endocrine system: an overview. Alcohol Health Res World. 1998;22(3):153-164.
- NCI Dictionary of Cancer terms. National Cancer Institute. https://www.cancer.gov/publications/dictionaries/cancer-terms. Accessed November 9, 2022.
- Forest MG. Sexual maturation of the hypothalamus: pathophysiological aspects and clinical implications. Acta Neurochir (Wien). 1985;75(1-4):23-42. https://doi.org/10.1007/BF01406321
- Batrinos ML. Testosterone and aggressive behavior in man. Int J Endocrinol Metab. 2012;10(3):563-568. https://doi.org/10.5812/ijem.3661
- Stephens MA, Wand G. Stress and the HPA axis: role of glucocorticoids in alcohol dependence. Alcohol Res. 2012;34(4):468-483.
- Gu S, Wang F, Cao C, Wu E, Tang YY, Huang JH. An Integrative Way for Studying Neural Basis of Basic Emotions With fMRI. Front Neurosci. 2019;13:628. Published 2019 Jun 19. https://doi.org/10.3389/fnins.2019.00628
- Aguilar-Raab C, Eckstein M, Geracitano S, et al. Oxytocin Modulates the Cognitive Appraisal of the Own and Others Close Intimate Relationships. Front Neurosci. 2019;13:714. Published 2019 Jul 16. https://doi.org/10.3389/fnins.2019.00714
- Government of Canada CIof HR. What is gender? what is sex? CIHR. https://cihr-irsc.gc.ca. Published April 28, 2020. Accessed November 9, 2022.
- Otto SP, Lenormand T. Resolving the paradox of sex and recombination. Nat Rev Genet. 2002;3(4):252-261. https://doi.org/10.1038/nrg761
- Svechnikov K., Soder O. Ontogeny of gonadal sex steroids. Best Practice & Research. Clinical Endocrinology & Metabolism. 2008;22(1):95โ106. https://doi.org/10.1016/j.beem.2007.09.002
- Bassett AJ, Ahlmen A, Rosendorf JM, Romeo AA, Erickson BJ, Bishop ME. The Biology of Sex and Sport. JBJS Rev. 2020;8(3):e0140. https://doi.org/10.2106/JBJS.RVW.19.00140
- Lauretta R, Sansone M, Sansone A, Romanelli F, Appetecchia M. Gender in Endocrine Diseases: Role of Sex Gonadal Hormones. Int J Endocrinol. 2018;2018:4847376. Published 2018 Oct 21. https://doi.org/10.1155/2018/4847376
- Hines M, Constantinescu M, Spencer D. Early androgen exposure and human gender development. Biol Sex Differ. 2015;6:3. Published 2015 Feb 26. https://doi.org/10.1186/s13293-015-0022-1
- Duke SA, Balzer BW, Steinbeck KS. Testosterone and its effects on human male adolescent mood and behavior: a systematic review. J Adolesc Health. 2014;55(3):315-322. https://doi.org/10.1016/j.jadohealth.2014.05.007
- Gropper SS, et al. Advanced Nutrition and Human Metabolism. Thomson Wadsworth; Belmont, CA: 2005
- Hooper DR, Kraemer WJ, Focht BC, et al. Endocrinological Roles for Testosterone in Resistance Exercise Responses and Adaptations. Sports Med. 2017;47(9):1709-1720. https://doi.org/10.1007/s40279-017-0698-y
- Dabbs JM Jr. Salivary testosterone measurements: reliability across hours, days, and weeks. Physiol Behav. 1990;48(1):83-86. https://doi.org/10.1016/0031-9384(90)90265-6
- Dabbs JM Jr, de La Rue D. Salivary testosterone measurements among women: relative magnitude of circadian and menstrual cycles. Horm Res. 1991;35(5):182-184. https://doi.org/10.1159/000181899
- Cooke PS, Nanjappa MK, Ko C, Prins GS, Hess RA. Estrogens in Male Physiology. Physiol Rev. 2017;97(3):995-1043. https://doi.org/10.1152/physrev.00018.2016
- Wolfrum R, Bordasch C, Holweg J. An endocrine model for the diagnosis of intrauterine growth retardation as demonstrated by the determination of total estrogen and pregnandiol 24-hour urinary excretion in 222 at-risk pregnancies. J Perinat Med. 1975;3(4):248-259.
- Enns DL, Tiidus PM. The influence of estrogen on skeletal muscle: sex matters. Sports Med. 2010;40(1):41-58. https://doi.org/10.2165/11319760-000000000-00000
- Yardley JE, Brockman NK, Bracken RM. Could Age, Sex, and Physical Fitness Affect Blood Glucose Responses to Exercise in Type 1 Diabetes? Front Endocrinol (Lausanne). 2018;9:674. Published 2018 Nov 15. https://doi.org/10.3389/fendo.2018.00674
- Olson BR. Exercise-induced amenorrhea. Am Fam Physician. 1989;39(2):213-221.
- Myklebust G, Maehlum S, Holm I, Bahr R. A prospective cohort study of anterior cruciate ligament injuries in elite Norwegian team handball. Scand J Med Sci Sports. 1998;8(3):149-153. https://doi.org/10.1111/j.1600-0838.1998.tb00185.x
- Waldรฉn M, Hรคgglund M, Werner J, Ekstrand J. The epidemiology of anterior cruciate ligament injury in football (soccer): a review of the literature from a gender-related perspective. Knee Surg Sports Traumatol Arthrosc. 2011;19(1):3-10. https://doi.org/10.1007/s00167-010-1172-7
- Chikani V, Ho KK. Action of GH on skeletal muscle function: molecular and metabolic mechanisms. J Mol Endocrinol. 2013;52(1):R107-R123. Published 2013 Dec 19. https://doi.org/10.1530/JME-13-0208
- Richter EA, Sylow L, Hargreaves M. Interactions between insulin and exercise. Biochem J. 2021;478(21):3827-3846. https://doi.org/10.1042/BCJ20210185
- Jensen J, Rustad PI, Kolnes AJ, Lai YC. The role of skeletal muscle glycogen breakdown for regulation of insulin sensitivity by exercise. Front Physiol. 2011;2:112. Published 2011 Dec 30. https://doi.org/10.3389/fphys.2011.00112
- American Diabetes Association. Blood sugar and exercise. Blood Sugar and Exercise | ADA. https://diabetes.org/. Accessed November 9, 2022.
- Park SW, Goodpaster BH, Strotmeyer ES, de Rekeneire N, Harris TB, Schwartz AV, Tylavsky FA, Newman AB. Decreased muscle strength and quality in older adults with type 2 diabetes: the health, aging, and body composition study. Diabetes 2006 1813โ1818. https://doi.org/10.2337/db05-1183
- https://eje.bioscientifica.com/view/journals/eje/181/6/EJE-19-0514.xml
- Tarnopolsky MA. Sex differences in exercise metabolism and the role of 17-beta estradiol. Medicine and Science in Sports and Exercise. 2008;40(4):648โ654.
- Trefts E, Williams AS, Wasserman DH. Exercise and the Regulation of Hepatic Metabolism. Prog Mol Biol Transl Sci. 2015;135:203-225. https://doi.org/10.1016/bs.pmbts.2015.07.010
- Paravati S, Rosani A, Warrington SJ. Physiology, Catecholamines. In: StatPearls. Treasure Island (FL): StatPearls Publishing; October 30, 2021.
- Jones CM 3rd, Greiss FC Jr. The effect of labor on maternal and fetal circulating catecholamines. Am J Obstet Gynecol. 1982;144(2):149-153. https://doi.org/10.1016/0002-9378(82)90616-0
- Dalmaz Y, Peyrin L. Sex-differences in catecholamine metabolites in human urine during development and at adulthood. J Neural Transm. 1982;54(3-4):193-207. https://doi.org/10.1007/BF01254929
- Horton TJ, Pagliassotti MJ, Hobbs K, Hill JO. Fuel metabolism in men and women during and after long-duration exercise. J Appl Physiol (1985). 1998;85(5):1823-1832. https://doi.org/10.1152/jappl.1998.85.5.1823
- Thau L, Gandhi J, Sharma S. Physiology, Cortisol. In: StatPearls. Treasure Island (FL): StatPearls Publishing; August 29, 2022.
- Wong SD, Wright KP Jr, Spencer RL, et al. Development of the circadian system in early life: maternal and environmental factors. J Physiol Anthropol. 2022;41(1):22. Published 2022 May 16. https://doi.org/10.1186/s40101-022-00294-0
- Zijlmans MA, Riksen-Walraven JM, de Weerth C. Associations between maternal prenatal cortisol concentrations and child outcomes: A systematic review. Neurosci Biobehav Rev. 2015;53:1-24. https://doi.org/10.1016/j.neubiorev.2015.02.015
- Mastorakos G, Ilias I. Maternal and fetal hypothalamic-pituitary-adrenal axes during pregnancy and postpartum. Ann N Y Acad Sci. 2003;997:136-149. https://doi.org/10.1196/annals.1290.016
- Yu T, Zhou W, Wu S, Liu Q, Li X. Evidence for disruption of diurnal salivary cortisol rhythm in childhood obesity: relationships with anthropometry, puberty and physical activity. BMC Pediatr. 2020;20(1):381. Published 2020 Aug 12. https://doi.org/10.1186/s12887-020-02274-8
- Moffat SD, An Y, Resnick SM, Diamond MP, Ferrucci L. Longitudinal Change in Cortisol Levels Across the Adult Life Span. J Gerontol A Biol Sci Med Sci. 2020;75(2):394-400. https://doi.org/10.1093/gerona/gly279
- Caplin A, Chen FS, Beauchamp MR, Puterman E. The effects of exercise intensity on the cortisol response to a subsequent acute psychosocial stressor. Psychoneuroendocrinology. 2021;131:105336. https://doi.org/10.1016/j.psyneuen.2021.105336
- Hill EE, Zack E, Battaglini C, Viru M, Viru A, Hackney AC. Exercise and circulating cortisol levels: the intensity threshold effect. J Endocrinol Invest. 2008;31(7):587-591. https://doi.org/10.1007/BF03345606
- Roa Dueรฑas OH, Koolhaas C, Voortman T, et al. Thyroid Function and Physical Activity: A Population-Based Cohort Study. Thyroid. 2021;31(6):870-875. https://doi.org/10.1089/thy.2020.0517
- Larson-Meyer DE, Gostas DE. Thyroid Function and Nutrient Status in the Athlete. Curr Sports Med Rep. 2020;19(2):84-94. https://doi.org/10.1249/JSR.0000000000000689
- Surks MI, Hollowell JG. Age-specific distribution of serum thyrotropin and antithyroid antibodies in the US population: implications for the prevalence of subclinical hypothyroidism. J Clin Endocrinol Metab. 2007;92(12):4575-4582. https://doi.org/10.1210/jc.2007-1499
- Kraemer WJ, Ratamess NA. Hormonal responses and adaptations to resistance exercise and training. Sports Med. 2005;35(4):339-361. https://doi.org/10.2165/00007256-200535040-00004
- Kraemer WJ, Marchitelli L, Gordon SE, et al. Hormonal and growth factor responses to heavy resistance exercise protocols. J Appl Physiol (1985). 1990;69(4):1442-1450. https://doi.org/10.1152/jappl.1990.69.4.1442
- Kraemer WJ, Fleck SJ, Dziados JE, et al. Changes in hormonal concentrations after different heavy-resistance exercise protocols in women. J Appl Physiol (1985). 1993;75(2):594-604. https://doi.org/10.1152/jappl.1993.75.2.594
- Goodwin ML. Blood glucose regulation during prolonged, submaximal, continuous exercise: a guide for clinicians. J Diabetes Sci Technol. 2010;4(3):694-705. Published 2010 May 1. https://doi.org/10.1177/193229681000400325
- Kjaer M. Adrenal medulla and exercise training. Eur J Appl Physiol Occup Physiol. 1998;77(3):195-199. https://doi.org/10.1007/s004210050321
- Copeland JL, Consitt LA, Tremblay MS. Hormonal responses to endurance and resistance exercise in females aged 19-69 years. J Gerontol A Biol Sci Med Sci. 2002;57(4):B158-B165. https://doi.org/10.1093/gerona/57.4.b158
- Consitt LA, Copeland JL, Tremblay MS. Hormone responses to resistance vs. endurance exercise in premenopausal females. Can J Appl Physiol. 2001;26(6):574-587. https://doi.org/10.1139/h01-032
- Razzak ZA, Khan AA, Farooqui SI. Effect of aerobic and anaerobic exercise on estrogen level, fat mass, and muscle mass among postmenopausal osteoporotic females. Int J Health Sci (Qassim). 2019;13(4):10-16.
- Hansen M. Female hormones: do they influence muscle and tendon protein metabolism? Proc Nutr Soc. 2018;77(1):32-41. https://doi.org/10.1017/S0029665117001951
- Klasson CL, Sadhir S, Pontzer H. Daily physical activity is negatively associated with thyroid hormone levels, inflammation, and immune system markers among men and women in the NHANES dataset. PLoS One. 2022;17(7):e0270221. Published 2022 Jul 6. https://doi.org/10.1371/journal.pone.0270221
- Ciloglu F, Peker I, Pehlivan A, et al. Exercise intensity and its effects on thyroid hormones [published correction appears in Neuro Endocrinol Lett. 2006 Jun;27(3):292]. Neuro Endocrinol Lett. 2005;26(6):830-834.
- Barari RA. Endurance training and ginger supplement on TSH, T3, T4 and testosterone and cortisol hormone in obese men. Iran J Basic Med Sci 2016;3:96โ103
- Krotkiewski M, Sjรถstrรถm L, Sullivan L, et al. The effect of acute and chronic exercise on thyroid hormones in obesity. Acta Med Scand. 1984;216(3):269-275. https://doi.org/10.1111/j.0954-6820.1984.tb03804.x
- Onsori M, Galdari M. Effects of 12 weeks aerobic exercise on plasma level of TSH and thyroid hormones in sedentary women. Eur J Sport Sci 2015;4:45โ9.